How technologically, economically, and environmentally feasible is a hydrogen economy in 2050?
- Fabrice Mattei
- Aug 5, 2022
- 16 min read
Fabrice Mattei
The initial thoughts on hydrogen economy began in 1972 when John O’M Bockris and Dr. John Appleby met at a hotel in Savoy and made notes on the tabletops which led to their article “The Hydrogen Economy – An Ultimate Economy” (Bockris, John O’.M 2013). Their paper discusses how hydrogen could take over from fossil fuel. Since then, hydrogen economy has fueled a great deal of interests and controversies. While some critics label hydrogen economy as illusionist, fashionable and wasteful[1], its proponents include hydrogen economy in their energy policy plans, companies like Siemens invest Euros 120 million in it[2] and patent applications for electrolysis water prosper.
This paper attempts to answer the question “how technologically, economically and environmentally feasible is a hydrogen economy in 2050?”. The primary aim is to analyze whether the production and storage capacities of hydrogen could address energy needs in a sustainable future at the net zero horizon of 2050. Then, the potential environmental implications in implementing hydrogen economy are explored with a focus on Europe through the highly debated concept of “additionality”. The paper concludes by summarizing the consequences of the arguments for or against the feasibility of hydrogen economy and point towards some other challenges than the ones covered in this study.
1. Hydrogen economy
Hydrogen economy describes a system that solves the environmental issues associated with the use of fossil fuels or Brown Hydrogen (“BH”) by shifting to Green Hydrogen (“GH”) as an energy carrier, and a storage medium (Bossel et al, 2006), (Crotogino, 2016).GH technology allows to convert electrical power to hydrogen via water electrolysis and utilizes it at scale (Fig.1). It helps overcoming the energy deficit created by fluctuating of Renewable Energy Sources (“RES”) since it can be stored for long periods either above the ground in sealed tanks or underground salt caverns (UNEP 2006).
[1] Keith Williams, “The Hydrogen Economy: The Picture is Confused, the Timeline is Too Long” available at https://seekingalpha.com/article/4400660-hydrogen-economy-picture-is-confused-timeline-too-long (Last visited on July 9, 2022). [2] Christophe Steitz, “Exclusive: Siemens spin-off tap hydrogen boom in wind alliance” January 12, 2021, Reuters, available at https://www.reuters.com/article/us-siemens-gamesa-r-siemens-energ-windpo-idUKKBN29I12Z (Lat visited on July 9, 2022).

Fig. 1 Hydrogen colour coding for various manufacturing processes. Green hydrogen is produced using renewable energy sources such as solar or wind energy, followed by water electrolysis. Grey and brown hydrogen are produced by methane steam reforming and coal gasifcation, respectively, and when combined with carbon capture and storage, blue hydrogen is produced. Turquoise hydrogen is produced through the pyrolysis of methane, with solid carbon as a by-product
Source: Osman et al., 2021
GH reduces, both directly and indirectly, greenhouse gas (“GHG”) emissions. Its storage capacity retains clean energy during overproduction and allows its reusing during shortage which avoid the need for flexible fossil-based energy production (Gabrielli et al., 2020). its applicability for different sectors such as transportation and heating and low carbon footprint make GH as a suitable driver for the energy transition (Ren et al., 2017).
2. Quantitative assessment
2.1 Energy demand needs
As of 2022 primary energy consumption needs are 620 quadrillion British thermal units (IEA 2021). Global energy demand is expected to increase by 47 % in the next 30 years due to population and economic growth. Although petroleum and other liquid fuels will remain the world’s largest energy source in 2050, RES will grow to nearly the same level (Chart 1.).

Chart 1 – Global primary energy consumption by energy source 2010-2050
Source: IEA 2021 available at https://www.eia.gov/outlooks/ieo/
Despite an increase of almost 300% since 1975 (Caspersen 2020), hydrogen, currently constitutes a very small portion of the global energy mix of approximately 90 Mt. it is met almost entirely (96.1%) by fossil fuel-based hydrogen responsible for almost 900 Mt of direct CO₂ emissions in 2020 or 2.5% of global CO₂ emissions in energy and industry units (Fig. 2). GH (3.9%) account for a small share of global production at 30 kt H2, water electrolysis and 16 Grey Hydrogen plants produced just 0.7 Mt H2 (IEA 2021).

ig. 2 Hydrogen production as % of Total Metric Tonnes as of 2022
Source: Balat M 2008.
2.2 Hydrogen market share projection by 2050
Climate change is widely acknowledged as a significant urgent threat by all 196 signatories the Paris Agreement on Climate Change[1]. By 2050, GHG emissions could be reduced by 5 to 6 gigatons annually
through the substitution of GH and BH (IRENA 2021). GH’s potentials are acknowledged through white papers, roadmaps of various governments (Cader et al, 2021), (Fig. 3). The EU Hydrogen Strategy of 2020 aims to increase the hydrogen’s role in Europe’s clean energy transition towards carbon neutrality and ”the priority for the EU is to develop renewable hydrogen, produced using mainly wind and solar energy” (EU Hydrogen Strategy 2020). [1] Paris Agreement on Climate Change, 2015 available at https://unfccc.int/sites/default/files/english_paris_agreement.pdf Last visited on July 7, 2022.

Fig 3 – timeline hydrogen-related government documents
Source: Cader et al, 2021
For hydrogen to contribute meaningfully to climate neutrality, it will account for 12% of final energy use by 2050 (IEA 2021), (IRENA 2021), (Talal at al., 2022), (Chart 2).

Chart 2 Share of total final consumption by fuel in the Bet Zero Emissions scenario 2020-2050
Source: IEA 2021 https://iea.blob.core.windows.net/assets/e57fd1ee-aac7-494d-a351-f2a4024909b4/GlobalHydrogenReview2021.pdf
its applicability for different sectors such as transportation and heating make it as a suitable driver for the energy transition (Chart 3).

Chart 3 - Hydrogen demand by sector in the Announced Pledges and Net Zero Emissions scenarios 2020-2050
Source: IEA 2021 https://iea.blob.core.windows.net/assets/e57fd1ee-aac7-494d-a351-f2a4024909b4/GlobalHydrogenReview2021.pdf
By 2050, global hydrogen production reaches 550 Mt H2, with 60% being GH 36% Grey hydrogen. This corresponds to global electrolyser capacity reaches 3,600 GW and the capture rate climbs to 1.5 Gt CO₂ /yr (IEA 2021), (Chart 4).
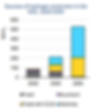
Chart 4 – Sources of hydrogen production in the Announced Pledges and Net Zero Emissions scenarios 2020-2050
Source: IEA 2021 https://iea.blob.core.windows.net/assets/e57fd1ee-aac7-494d-a351-f2a4024909b4/GlobalHydrogenReview2021.pdf
In conclusion, hydrogen economy is projected to represent 12 % share of final energy in 2050 with almost 2/3rd from GH. However, there are major uncertainties such as the pace of innovation, production volume, international governance, geopolitical change, prioritization of sustainability and climate, electricity costs (Bohm et al, 2020). They all make projections difficult and will be addressed in the next qualitative assessment.
3. Qualitative assessment
To assess the feasibility of Hydrogen economy the knowledge of the current and projected improvement of technologies (3.1), development of costs in manufacturing and storage of GH (3.2) and environmental impacts are mandatory (3.3) (The Hydrogen Economy 2004), (Muradov et al, 2005) (Schmidt et al, 2019), (Bohm et al. 2019), (Dehghanimadvar et al, 2020), (UK 2021), (Mayyas et al., 2022). These three factors are interconnected and should be considered together. The faster those improvements occur the sooner GH will become economically viable. Also, taxes or GHG emissions costs rise and social acceptance of GH (Scott et al. 2020), will contribute making hydrogen economy feasible but are not covered in this essay.
3.1 Innovations factor
How to forecast with accuracy technology improvements and cost competitiveness for GH technologies while they are relatively immature? This is a major challenge as highlighted in Bohm et al. 2019:
“Due to the novelty of the technology and therefore low numbers of real implementations, availability of data on cumulative productions and production costs is limited”
which we attempt to address by combing patent data with technology forecasting as a proxy to technology learning effect.
(i) Patent data
One of the advantages of using patent data in this analysis is its undisputed and purity status (Baumann et al, 2021). In 2017 the total number of patent families covering GH production processes overtook those based on hydrocarbon sources (EPO & IRENA 2022), (WIPO 2022). This shift reaffirms the priority given to research on GH and continuous growth of RES and their integration with GH (Chart 5).

Chart 5 – Worldwide patent filings for green hydrogen technologies
Source : (EPO & IRENA 2022
Furthermore, the sustained growth of granted patents illustrates the quality and potentials for use of technological improvements since only patents complying with patentability requirements are ultimately granted (WIPO 2022), (Chart 6).

Chart 6 – Worldwide granted patents for green hydrogen technologies
The chart illustrates the trend of granted patent applications, both in net values
(darker colour) and in cumulative values (lighter colour)
Source : (EPO & IRENA 2022
Since the patent data is a rather static indicator for technological evolution, other tools looking at the forecasting and diffusion of GH technologies in a dynamic angle shall also be considered.
(i) Technology forecasting
Technology forecasting helps identifying the opportunities and challenges of technology orientations and diffusion. Data collected confirm interests for GH while highlighting low-medium technology readiness level (“TRL”) and divergences between GH technologies varying from mature (AEC) over commercial (PEM) down to demonstration (SOEC) stage (Schmidt et al., 2017). Despite deficiencies in GH technologies, improvement and development in processes and materials can accelerate their path to the market (Dehghanimadvar et al, 2020), (Charts 7a & 7b).

7a Hydrogen Production Technologies Acceleration 7b Technology readiness Level for hydrogen
Charts 7a & 7b
Source: Dehghanimadvar et al, 2020
However, since patent families are mostly originating from 10 industrialized countries (Chart 8) and concentrates within private entities this raises the question of their transfer, diffusion and access both for developing countries in need for new technology and for industrialized countries, in order to allow hydrogen economy to prosper.

Chart 8 – Worldwide patent filings for green hydrogen technologies by countries
Source : (EPO & IRENA 2022)
To determine the extent of the transfer and access to GH technologies, a review of Clean Development Mechanism’s projects on hydrogen from 1997-2021 was conducted and only two projects dated 2007 and 2009 were found between Japan and Indonesia and India[1]. According to Hydrogen Council here are 228 large-scale hydrogen projects of a value element of USD 70 billion across 30 developed and developing countries also underway.
Few studies have investigated the level of diffusion of GH technologies. Correia et al, 2022 list the factors influencing their diffusion by using six cases studies including large ones such as H2 Future (Austria) and NortH2 (Netherlands). The publication concludes that the factors influencing more negatively the diffusion of GH are the degree of certification, degree of complexity on the innovation, the ability of the user or recipient of the technologies (Table 1).
[1] Clean Development Mechanism database of projects is available at https://cdm.unfccc.int/Projects/projsearch.html Last visited on July 8, 2022.

Table 1 – Influencing factors on transfer of Hydrogen technologies
Source: Correia et al, 2022
While patent data and technology forecasting predict growth in GH technologies, their current TRL, complexity, and exclusive ownership within a few companies could lock out other competitors and countries where hydrogen access is most needed (Fig. 3) and result in slowing down their diffusion and failing to deliver a global hydrogen economy (Ludwig et al, 2021).

Fig 3. Hydrogen consumption in 2020 (million tonnes per year)
Source: IRENA 2022 b
3.2 Economic factor
How to displace and reduce the role currently met by fossil fuels at a price of hydrogen as low as USD 0.8-1/kg, whereas GH using electrolysis is currently produced at much higher price? As discussed above, since GH technologies are rather in their infancy, their total investment (“CAPEX”) and maintenance (“OPEX”) costs are too burdensome to be competitive in commercial applications. Under what conditions could GH be economically competitive against BH and Grey Hydrogen by 2050? Several publications have pointed out the main drivers of cost reductions as technological learning, economies of scale (Tschuiya et al 2004), (Lee et al., 2019), (Assessment Report 2020) (Bohm et al 2019), (Bristowe et al, 2021), (IRENA 2019), (UK 2021), electricity cost and automation level. In this paper, levelized cost of hydrogen (“LCOH”) and levelized cost of Storage (“LCOS”) are used as proxies to cost reductions assessment to arrive at a thorough approach of electroliers’ value chain.
(i) LCOH
The LCOH is the ratio of CAPEX and OPEX costs divided by the actual amount of hydrogen produced. It is expressed as a cost (e.g., USD) per energy unit (e.g., MWh) of hydrogen produced. When compared to a 2019 baseline (Bohm, et al 2018), (Hydrogen Council 2021) (IRENA 2019) consider that cost reduction by 2050 could more than ×100-fold. However, this requires deeper investigation on the factors influencing such cost reduction (Chart 9).

Chart 9 - Historical and projected cost reductions across a range of net-zero energy technologies
as a function of installed capacity relative to a 2019 baseline.
Source: Bristowe et al., 2021
a) Economies of scale
Most publications focus on PEM electrolysis due to its maturity level and potentials. UK 2021 predicts a 7% cost reduction per doubling in installed global capacity while AEC, as a more mature technology, carries lower expected cost reduction. Bristowe et al, 2021 reached a similar conclusion and found that the total capital cost of a PEM electrolysis system can be reduced by 70% as a result of volume manufacturing. However, once economies of scale have been achieved (>300 units), the CAPEX is dominated by the Balance of Plant (Chart 10).

Chart 10 – Cost reduction level for PEM
Source: Bristowe et al., 2021
b) Learning curve
The learning curve captures the experience expressed in cumulative production of the one product or technology (Sondes et al., 2008). Whilst there are different methodologies for assessing learning curves, most of them appear too simplified and generic. Bohm et al. 2019 propose a rather innovative approach by assigning learning rate (“LR”) to all components of GH technologies, through the lens of costs, but they fail to precisely substantive the actual learnings which can be transferred (Table 2).

Table 2 – Cost allocation and learning rate percentage
Source: Bohm et al. 2019
Similarly, other publications give general percentage with varying uncertainty level (Table 3).

Table 3 : Summary table of learning curve percentage from various publications
Source: essay’s author
To substantive data on the learning curve factor, we propose to complement Bohm et al. 2019 by harvesting and assigning learnings from patent data to GH technologies components as summarized below (Table 4).

Table 4 : Summary table of learnings from patent data for green hydrogen
Source: essay’s author using data collected from (EPO & IRENA 2022)
c) Electricity cost
In most studies the cost of hydrogen from electrolysis is mainly driven by the use and price of electricity as illustrated by Bristowe et al, 2021 (Chart 11).

Chart 11 – Cost estimate reduction of green hydrogen
Source: Bristowe et al, 2021
While using electricity from the grid, from dedicated electricity generation sources or from curtailment will result in different pricing and cost reduction scenarios as shown in Chart 12a and 12b although PEM using dedicated RES won’t be as cost competitive as if it uses grid electricity. This is particularly relevant in the context of EU policy framework where the principle of additionality won’t allow GH projects to use grid electricity except when surplus are available.

Chart 12a - LCOH estimates for electrolysis technologies, connected to different electricity sources, commissioning from 2020 to 2050, £/MWh H2 (HHV)
Source: UK 2021

Chart 12 b - Comparison of LCOH estimates across different technology types at central fuel prices commissioning from 2020 to 2050, £/MWh H2 (HHV)
Source: UK 2021
(II) LCOS
While assessing the amount of hydrogen produced is covered by LCOH, for evaluating LCOS, the amount of hydrogen stored is assessed and the unit is kg. Schmidt et al., 2019 use the LCOS to estimate the discounted cost per unit of discharged electricity for a specific storage technology (e.g. GH) and application (e.g. seasonable storage). Their calculation accounts for all technical and economic factors affecting the lifetime cost of discharging stored electricity. The main factors that impact the LCOS are nominal power capacity, discharge duration which are CAPEX based and annual cycles, and electricity cost which are OPEX dependent. For seasonal storage with more than 700 h discharge, which is one of GH main applications, GH is likely to become most cost efficient with a projected lowest lifetime costs reduction by 53% in 2050.
The combination of LCOH and LCOS show opportunities for production and storage cost reduction. The distribution cost reduction of GH is still unknown as different options are being considered including retrofitting pipelines. The lack of data and nascent volume of GH projects make cost reductions projections challenging. The combination of techno-economic factors gives more certainty to cost trajectories and reduce assumptions. Since electricity cost is one the main production and storage cost factor of GH, what would it be in 2050 and how would it impact GH’s environmental footprint? In this regard, the EU draft directive offers an interesting perspective[1].
3.3 Environmental footprint
By 2050, GH and BH production is forecasted to generate over 500 Mt H2 thus enable hydrogen-based fuels to avoid up to 60 Gt CO₂ emissions from now until 2050 amounting 6.5% of total cumulative GHG emissions reductions in a net zero scenario. Electrolysis has the smallest water impact, using approximately 9 kg H2O/kg H2 of hydrogen while Grey Hydrogen use 13-18 kg H2O/kg H2 and BH uses 40-85 kg H2O/kg H2[2]. However, the environmental concern isn’t just about water. With almost all of the EU’s hydrogen is made from fossil gas, to meet 13-14% of energy demand with hydrogen in 2050 in a 1.5 degree scenario of climate change (EU Hydrogen Strategy 2020) hydrogen will necessitate extensive use of RES (Chart 13). There is a risk that RES would stop contributing to the grid decarbonization and be diverted to meet the ambitious but still modest 2030 hydrogen target of 13-14 %. The additionality principle provided in the draft Delegated Act aims to avoid that risk. Under the additionality, electricity power must be generated from RES built specifically to produce GH and are additional to the existing renewal energy installations. The only exception is that GH can be produced from electricity surplus that otherwise would have gone to waste. In theory, this principle addresses a major environmental risk by preventing hydrogen companies from diverting RE from existing sources and be replaced by fossil electricity to fill the gap, and thereby increase emissions. It may also contribute to electricity cost reduction due to an increase amount of RES installations.
[1] The first and second draft Delegated acts clarifying EU rules applicable to renewable hydrogen under the 2018 Renewable Energy Directive are available at https://ec.europa.eu/info/news/commission-launches-consultation-regulatory-framework-renewable-hydrogen-2022-may-20_en Last visited on July 7, 2022. [2] Herib Blanco, “Hydrogen production in 2050: how much water will 74EJ need?” July 22, 2021 https://energypost.eu/hydrogen-production-in-2050-how-much-water-will-74ej-need/ Last visited on July 9, 2022.

Chart 13 – Extra renewal energy sources needed in Europe to support the growth of hydrogen from 2020-2050
However, critics argue that the lead time for investments into some RE installations especially offshore wind take almost a decade and fail to coincide with the much shorter time period needed to construct and operate and electrolyser. Hydrogen companies raise the risk that until dedicated electricity capacity is made available, GH project developers will have a very low incentive to build them. This put at risk the development of hydrogen economy in the EU (Hydrogen Europe 2021).
Conclusion
The combination of techno-economic and environmental factors led to support the expansion and cost reduction of GH in the next 30 years. Would that be sufficient for hydrogen economy to overtake Fossil fuel economy? probably yes, however electron economy is likely to dominate the energy ecosystem by that time. Other considerations including retrofitting or construction of supply infrastructure for hydrogen distribution and consumers acceptance that hydrogen is economical, practical, and safe shall also be considered. With a projected modest 12 % market share power generation in 2050, the transition to a hydrogen economy is feasible but it is likely to be gradual with a high level of subsidies and policy intervention in view of gas supply shortage from Russia.
--------------------
Bibliography
----------------------------------------------------------
Assessment Report 2020 - Assessment of Hydrogen Production Costs from Electrolysis: United States and Europe International Council on Clean Transportation 2020 available at https://theicct.org/wp-content/uploads/2021/06/final_icct2020_assessment_of-_hydrogen_production_costs-v2.pdf last visited on July 9, 2022.
Balat, M 2008 - “Potential importance of hydrogen as a future solution to environmental and transportation problems,”International Journal of Hydrogen Energy Volume 22, Issue 15 August 2008, Pages 4013-4029.
Baumann et al, 2021 - Baumann, Manuel et al. “Comparative Patent Analysis for the Identification of Global Research Trends for the Case of Battery Storage, Hydrogen and Bioenergy.” Technological forecasting & social change 165 (2021): 120505–. Web.
Bockris, John O’.M 2013 - “The Hydrogen Economy: Its History.” International journal of hydrogen energy 38.6 (2013): 2579–2588. Web.
Bohm et al. 2018 - Böhm, H.; Zauner, A.; Goers, S.; Tichler, R.; Kroon, P. Innovative Large-Scale Energy Storage Technologies and Power-to-Gas Concepts after Optimization. 2018. Available online: https://cordis.europa.eu/project/id/691797 Last visited on July 8, 2022
Bohm et al, 2019 - Böhm, Hans, Sebastian Goers, and Andreas Zauner. “Estimating Future Costs of Power-to-Gas – a Component-Based Approach for Technological Learning.” International journal of hydrogen energy 44.59 (2019): 30789–30805. Web.
Bohm et al, 2020 - Böhm, Hans et al. “Projecting Cost Development for Future Large-Scale Power-to-Gas Implementations by Scaling Effects.” Applied energy 264 (2020): 114780–. Web.
Bossel et al, 2006 - Bossel, Ulf. “Does a Hydrogen Economy Make Sense?” Proceedings of the IEEE 94.10 (2006): 1826–1837. Web.
Cader et al, 2021 - Cader, Justyna, Renata Koneczna, and Piotr Olczak. “The Impact of Economic, Energy, and Environmental Factors on the Development of the Hydrogen Economy.” Energies (Basel) 14.16 (2021): 4811–. Web.
Caspersen 2020 – Michael Caspersen Hydrogen is already a well-established market, but currently based on fossil feedstock, KPMG available at https://home.kpmg/xx/en/home/insights/2020/11/the-hydrogen-trajectory.html last visited on July 7, 2022
Correia et al, 2022 - Speed of Innovation Diffusion in Green Hydrogen Technologies Lourenço Correia(B) , Oliver Schwabe(B) , and Nuno Almeida(B) o Springer Nature Switzerland AG 2022 J. O. P. Pinto et al. (Eds.): 15th WCEAM Proceedings, LNME, pp. 101–111, 2022. https://doi.org/10.1007/978-3-030-96794-9_10
Crotogino et al., 2016 - Crotogino, Fritz. “Larger Scale Hydrogen Storage.” Storing Energy - With Special Reference to Renewable Energy Sources. Elsevier, 2016. 411–429. Prin
Dehghanimadvar et al, 2020 - Dehghanimadvar, Mohammad et al. 2020 - “Hydrogen Production Technologies: Attractiveness and Future Perspective.” International journal of energy research 44.11 (2020): 8233–8254. Web.
EPO & IRENA 2022 - Francesco Pasimeni (IRENA) Ufuk Sezer (IRENA) Francisco Boshell (IRENA) Geert Boedt (EPO) Innovation trends in electrolysers for hydrogen production published and edited by European Patent Office (EPO) and International Renewable Energy Agency (IRENA) 2022
EU Hydrogen Strategy 2020- A hydrogen strategy for a climate-neutral Europe – European Commission, July 8, 2020 available at https://ec.europa.eu/energy/sites/ener/files/hydrogen_strategy.pdf last visited on July 7, 2022
Gabrielli et al, 2020 - Gabrielli, Paolo et al. “Seasonal Energy Storage for Zero-Emissions Multi-Energy Systems via Underground Hydrogen Storage.” Renewable & sustainable energy reviews 121 (2020): 109629–. Web.
Hydrogen Council 2021 – Hydrogen Council in Collaboration with McKinsey & Company. Hydrogen Insights: A Perspective on Hydrogen Investment, Market Development and Cost Competitiveness. 2021. Available online: https://hydrogencouncil.com/wp-content/uploads/20 21/02/Hydrogen-Insights-2021.pdf Last visited on July 6, 2022.
Hydrogen Europe 2021 - A workable approach to additionality, geographic and temporal correlation is key to the achievement of the EU Hydrogen Strategy, June 2021, available at https://hydrogeneurope.eu/wp-content/uploads/2021/11/2021.06-Hydrogen-Europe_Addionality-Position-Paper.pdf Last visited on July 9, 2022
IEA 2021 - International Energy Outlook 2021 Global Hydrogen Review 2021 International Energy Agency, US Energy Information Administration. Available at: https://www.eia.gov/outlooks/ieo/ last visited on July 7, 2022.
IEA. 2019 - The Future of Hydrogen. Available at: https://webstore.iea.org/the-future-of-hydrogen last visited on July 7, 2022.
IRENA 2019 – Global Energy Transformation : Roaddmap to 2050, International Renewable Energy Agency
IRENA 2022 - Global Hydrogen Trade To Meet The 1.5°C Climate Goal 2022.
IRENA 2022 b) - Irena Geopolitics of the Energy Transformation The Hydrogen Factor available at https://www.irena.org/publications/2022/Jan/Geopolitics-of-the-Energy-Transformation-Hydrogen last visited on July 10, 2022
Lee et al., 2019 - Boreum Lee, Hyujun Lee, Hyun-Seok Cho, Won-Chi Cho, Chang Hee Kim and Hanknwon Lim “Projected economic outlook and scenario analysis for H2 production by alkaline water electrolysis on the basis of the unit electricity price, the learning rate, and the automation level” Sustainable Energy & Fuels, Royal Society of Chemistry 2019 Volume 3, 1799-1807.
Ludwig et al, 2021 - Max Ludwig, Martin Lüers, Markus Lorenz, Esben Hegnsholt, Minjee Kim, Cornelius Pieper, and Katharina Meidert The Green Tech Opportunity in Hydrogen April 12, 2021, The Boston Consulting Group.
Mayyas et al., 2022 - Manufacturing Cost Analysis for Proton Exchange Membrane Water Electrolyzers Ahmad Mayyas, Mark Ruth, Bryan Pivovar, Guido Bender, and Keith Wipke National Renewable Energy Laboratory
Muradov et al, 2008 - Muradov, Nazim Z, and T. Nejat Veziroğlu. “‘Green’ Path from Fossil-Based to Hydrogen Economy: An Overview of Carbon-Neutral Technologies.” International journal of hydrogen energy 33.23 (2008): 6804–6839. Web.
Muradov et al., 2005 - Muradov et al, 2005 - Muradov, N.Z, and T.N Veziroǧlu. “From Hydrocarbon to Hydrogen–carbon to Hydrogen Economy.” International journal of hydrogen energy 30.3 (2005): 225–237. Web.
Osman et al, 2021 - Osman , A. I., Mehta, N., M. Elgarahy, A., Hefny, M., Al-Hinai, A., Al-Muhtaseb, A. H., & Rooney, D. W. (2021). Hydrogen production, storage, utilisation and environmental impacts: a review. Environmental Chemistry Letters. https://doi.org/10.1007/s10311-021-01322-8
Ren et al., 2017 - Ren, Jingzheng et al. “Opportunities and Future Challenges in Hydrogen Economy for Sustainable Development.” Hydrogen Economy : Supply Chain, Life Cycle Analysis and Energy Transition for Sustainability. N.p., 2017. 277–305.
Schmidt et al, 2017 - Schmidt, O et al 2017 - “Future Cost and Performance of Water Electrolysis: An Expert Elicitation Study.” International journal of hydrogen energy 42.52 (2017): 30470–30492. Web.
Schmidt et al, 2019 - Schmidt, Oliver et al. “Projecting the Future Levelized Cost of Electricity Storage Technologies.” Joule 3.1 (2019): 81–100. Web
Schoots et al, 2010 - Schoots, K, G.J Kramer, and B.C.C van der Zwaan. “Technology Learning for Fuel Cells: An Assessment of Past and Potential Cost Reductions.” Energy policy 38.6 (2010): 2887–2897. Web.
Scott et al. 2020 - Scott, Matthew, and Gareth Powells. “Towards a New Social Science Research Agenda for Hydrogen Transitions: Social Practices, Energy Justice, and Place Attachment.” Energy research & social science 61 (2020): 101346–. Web
Sondes et al., 2008 - Kahouli, Sondès. “Technological Learning in Energy–environment–economy Modelling: A Survey.” Energy policy 36.1 (2008): 138–162. Web.
Talal at al., 2022 - Talal Yusaf et al. “Hydrogen Energy Demand Growth Prediction and Assessment (2021–2050) Using a System Thinking and System Dynamics Approach.” Applied sciences 12.2 (2022): 781–. Web
The Hydrogen Economy 2004 - The hydrogen economy opportunity, costs, barriers and R&d needs - Committee on Alternatives and Strategies for Future Hydrogen Production and Use Board on Energy and Environmental Systems Division on Engineering and Physical Sciences 2004
Tsuchiya et al, 2004 - Tsuchiya, Haruki, and Osamu Kobayashi. “Mass Production Cost of PEM Fuel Cell by Learning Curve.” International journal of hydrogen energy 29.10 (2004): 985–990. Web.
UK 2021 - Hydrogen Production Costs 2021, Department for Business, Energy and Industrial Strategy, August 2021 available at https://www.gov.uk/government/publications/hydrogen-production-costs-2021
UNEP 2006 - The Hydrogen Economy A Non-Technical Review 2006, United Nations Environment Programme.
WIPO 2022 - Patent Landscape Report, Hydrogen Fuel Cells in Transportation, World Intellectual Property Organization.